The light of a supernova that has traveled for 10 billion years to reach us has given us a new measurement of the Hubble constant – the accelerating rate at which the Universe is expanding.
Called SN H0pe, it’s one of the most distant Type Ia supernovae we’ve ever seen, and measurements of the rate at which it seems to be receding have given a Hubble constant of 75.4 kilometers per second per megaparsec.
This leaves us in a dilly of a pickle. Measures of the early Universe based on a different method referred to as a ‘standard ruler’ tend to return slower results of around 67 kilometers per second per megaparsec.
While SN H0pe appears as it did a whole 4 billion years after the Big Bang, it’s much further back in time than other ‘standard candle‘ measurements taken in the nearby Universe, which are around 73 kilometers per second per megaparsec – suggesting that the tension is consistent throughout the visible Universe, as far as we can see.
This takes one possible explanation for the tension off the table: that local space is receding at a higher rate than distant space. If one technique gets the same results for both the distant and local Universe, that suggests that H0 is more or less uniform.
frameborder=”0″ allow=”accelerometer; autoplay; clipboard-write; encrypted-media; gyroscope; picture-in-picture; web-share” referrerpolicy=”strict-origin-when-cross-origin” allowfullscreen>
OK, we can explain. The whole problem is this thing called the Hubble tension – an unresolved discrepancy between the results of different methods used to measure the accelerating expansion of the Universe.
The standard ruler approach uses relics of the early Universe. These are things like the cosmic microwave background, or fossilized densities in the distribution of galaxies called baryon acoustic oscillations.
Standard candles, on the other hand, are objects of known intrinsic brightness, such as Cepheid variable stars, and Type Ia supernovae. Since these objects are presumed to emit a relatively consistent amount of light, we can work out how far away they are by measuring their apparent brightness.
But their usefulness is limited by their distance – at some point, they become too far away to see, so they’re typically only used to measure the Hubble constant in the local Universe.
H0pe is a lot farther than most Type Ia supernovae we can see. That’s because it’s magnified and triplicated by a quirk of space-time known as a gravitational lens.
Around a massive object, such as a galaxy or galaxy cluster, space-time tends to curve; any light traveling through this curvature can be repeated and magnified, much like curved glass magnifies whatever is behind it.
H0pe, as we explained last year when the discovery was made, sits behind a galaxy cluster. As the light from the supernova traveled through the gravitational lens generated by the cluster, it magnified and split into three distinct dots.
“This is similar to how a trifold vanity mirror presents three different images of a person sitting in front of it. In the Webb image, this was demonstrated right before our eyes in that the middle image was flipped relative to the other two images, a ‘lensing’ effect predicted by theory,” says cosmologist Brenda Frye of the University of Arizona.
“To achieve three images, the light traveled along three different paths. Since each path had a different length, and light traveled at the same speed, the supernova was imaged in this Webb observation at three different times during its explosion.
“In the trifold mirror analogy, a time-delay ensued in which the right-hand mirror depicted a person lifting a comb, the left-hand mirror showed hair being combed, and the middle mirror displayed the person putting down the comb.”
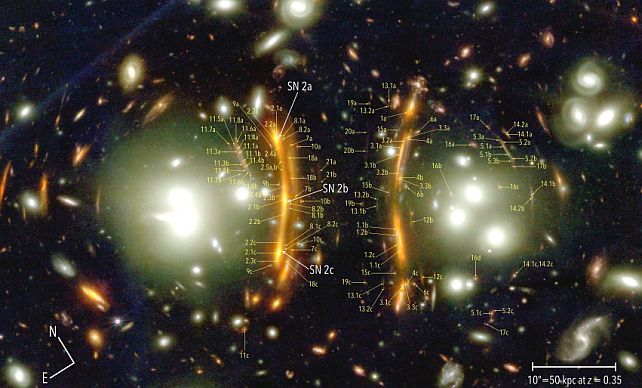
This allowed the researchers to make a detailed measurement of the Hubble constant in the distant Universe using a standard candle technique usually only applied to the local Universe. The result of 75.4 kilometers per second per megaparsec may not resolve the tension, but it does narrow down what the explanation might be.
The Hubble tension is one of the biggest problems in cosmology. It’s not remotely trivial: it will tell us how big and old the Universe is, and give us more accurate measurements across space-time as a whole.
Astronomers generally use a Hubble constant of around 70 kilometers per second per megaparsec to determine distances to cosmic objects – which is just an estimate based on the best data we currently have.
Resolving the Hubble tension will likely be a Nobel-winning achievement. And the good news is that we seem to be getting closer.
Gravitational waves have given us a new tool to try and narrow it down – the standard siren. Standard siren measurements have been made; they’re in the vicinity of both standard rulers and standard candles, so still inconclusive, but it’s only a matter of time now.
And a few more observations from the JWST could get us there. With just four more events like H0pe, the confidence level of the measurement could be improved to over three sigma. That will be a good day.
The report of the new measurement has been submitted to The Astrophysical Journal, and is available on preprint server arXiv.