In a feat of spectacular ingenuity, scientists have for the first time managed to capture on film a microscopic shock wave traveling through a single human cell.
Using a system called a spectrum circuit, a team led by engineer Takao Saiki of the University of Tokyo has achieved both the nanoscale resolution and the speed required to capture, frame by frame, the movement of an underwater acoustic shock as it passes through a cultivated HeLa cell, from beginning to end.
The resulting images reveal new information about how shocks interact with biological cells, and the breakthrough also offers an avenue for understanding shockwave therapy, and characterizing cells based on their acoustic properties.
“For the first time in history, as far as we know, we have directly observed the interaction between a biological cell and a shock wave, and experimentally demonstrated that the velocity of the shock wave propagating inside the cell is faster than the outside of the cell,” Saiti explains.
“Furthermore, our approach has enabled us to demonstrate high-speed photography across a wide time range, which includes picosecond (one-trillionth of a second), nanosecond (one-billionth of a second) and millisecond (one-thousandth of a second) timescales.”
Taking images of single cells is difficult. They’re very fragile and easily damaged, for a start. And then they’re tiny, requiring extremely high-resolution capabilities. Because they’re so small, it also doesn’t take very long for something to move from one side of the cell to the other. If you blink, you will actually miss it.
So any system designed to image shockwave propagation through a cell needs to tick several boxes. It must function on incredibly tiny scales, both spatially and temporally. And it needs to be able to do so without damaging the cell.
The spectrum circuit is an optical circuit that runs on light, rather than electricity. The team uses this to generate laser pulses that won’t damage the cell, sending them out into a cell sitting in a dish of water at different nanosecond intervals.
Then, they used an imaging technique called sequentially timed all-optical mapping photography, or STAMP, which uses burst photography to generate a series of sequential images on short timescales.
This camera was used to photograph the wave as it traveled through the cell, capturing frames at intervals of 1.5 nanoseconds, and an exposure time for each frame of 44 picoseconds. The results clearly show the wavefront as it moves from one side of the cell to the other.
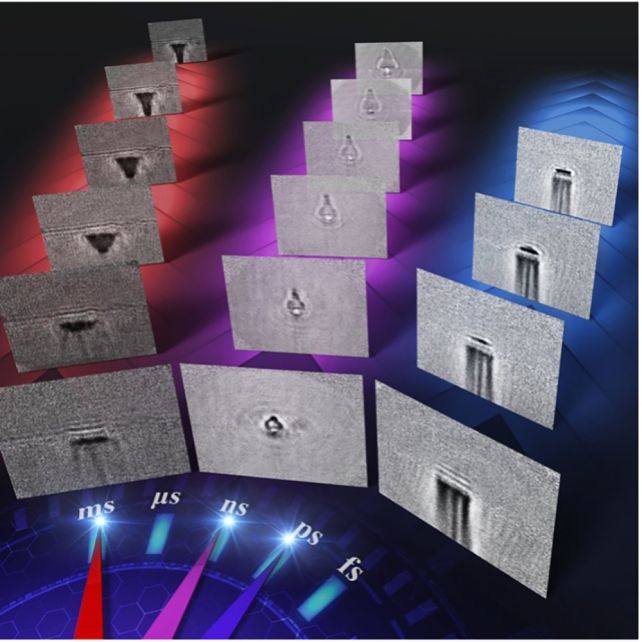
The technology doesn’t just have applications in biological imaging. The researchers also used it to image the effects of laser ablation on glass.
They fired femtosecond (one-quadrillionth of a second) laser pulses at a glass sheet, and were able to observe in detail the process of the laser hitting the glass, the shockwave, and ablation, over different time scales.
The technology, the team says, gives scientists a new tool for understanding microscopic, fast interactions, breaking them down into a series of processes that can help develop better tools for controlling them. This has wide-ranging implications.
“Our technology provides opportunities to reveal useful but unknown high-speed phenomena by enabling us to observe and analyze such ultrafast processes,” says engineer Keiichi Nakagawa of the University of Tokyo.
“Next, we are planning to use our imaging technique to visualize how cells interact with acoustic waves, like those used in ultrasound and shock wave therapy. By doing this, we aim to understand the primary physical processes that activate subsequent therapeutic effects in the human body.”
The research has been published in Science Advances.